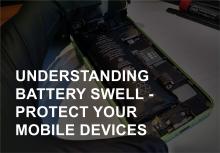
Mobile devices are designed to survive crammed into our pockets day-in and day-out; they can even survive toddlers and teenagers for a few months. Yet placing thousands of phones or tablets into server racks can actually be a harder place for these devices to survive long term. The continuous battery charging, high processor usage, and high temperatures present in test labs racks present unique challenges to device longevity since they were not designed for these applications.
The most prominent failure mechanism under these conditions is battery swelling. If left unchecked, these devices may undergo RUD: rapid unplanned disassembly. Obviously such an event can be catastrophically expensive and even dangerous.
In order to mitigate battery swell in device labs, it is useful to understand the underlying causes of the issue. With an understanding of the fundamentals, engineers can design and implement systems and processes which reduce battery stressors in device-lab environments. Avoiding battery stress will reduce unplanned outages and expensive device replacements.
Lithium Battery Chemistry
Video conferencing has the capacity to make a greater impact than ever before, as we rely on screens to mediate our face-to-face interactions. Why use the Acroname USBHub3+ in your conference room setup? Here are some of the benefits of using USBHub3+:
Electrochemical batteries are constructed of two electrodes, a cathode and an anode, separated by an electrolyte. Normally the electrodes are named cathode or anode based on what type of reaction happens at each site. With lithium-based cells, both electrodes act as an anode or cathode if the cell is charging or discharging. As such, it is more accurate to define the electrodes by their relative potentials: positive-electrode and negative-electrode (ref: “Lithium ion battery degradation: what you need to know”).
Almost all modern mobile devices use “lithium-ion” (Li+) or “lithium polymer” (LiPO) electrochemical batteries, and there is a variety of chemistries used to make these cells.
The specific chemistries used in the battery cell determine the cell’s power density, energy density, and, most notably for this investigation, failure mechanisms. The negative-electrode in lithium based cells is some form of lithiated carbon graphite or another form of carbon lattice, such as silicon-carbon. The positive-electrode is a metal oxide. Between the electrodes is an electrolyte comprising a lithium salt in an organic solvent or polymer.
In addition to the electrodes and electrolyte, these batteries comprise various separators, current collectors and housing materials. Some of these additional components play a role in preventing failures but also contribute negatively to the damage after a loss occurs.
Lithium-Ion Batteries
The positive-electrode in lithium-ion batteries is usually lithium cobalt oxide (LiCoO2) or lithium manganese oxide (LiMn2O4 or Li2MnO3). Variations which include nickel (LiNiMnCoO2 or NMC) and other metals are starting to be used as these structures may reduce capacity degradation over charge cycles. Iron containing positive-electrodes (LiFePO4) are also common for improved temperature stability, cycle-life, and power density. These positive-electrode materials all form lattices or matrices which allow lithium ions to migrate in and out of the structure during charging and discharging.
The lithium ions then migrate through the electrolyte into the negative-electrode structure. The lithium-ion battery’s electrolyte distinguishes it from LiPo cells. A lithium-ion cell’s electrolytes are generally a lithium salt such as LiPF6 or LiClO4 in an organic solvent. This solvent must be non-aqueous since lithium reacts violently when exposed to water, even humidity in the air. Typically, these solvents are organic carbonates such as ethylene carbonate (EC), dimethyl carbonate (DMC), diethyl carbonate (DEC), propylene carbonate (PC), or a combination of these. These organic solvent mixtures are almost all highly flammable; e.g.: DMC has a flash point around 17˚C.
In part due to the liquid electrolyte form, Li+ batteries are most commonly found in rigid cylindrical metal housings.
Lithium-Polymer Batteries
LiPo batteries use the same LiPF6 as the active electrolytic salt, but instead of being dissolved in a liquid organic solvent, they use a solid polymer electrolyte such as poly(ethylene oxide), poly(acrylonitrile), poly(methyl methacrylate) or poly(vinylidene fluoride). While the polymer electrolyte of LiPo batteries is commonly thought of as a solid, it sometimes takes a gel-like form; i.e. it is more correctly just not a liquid like the electrolyte in Li+ cells.
The gel-like form of the electrolyte used in LiPo batteries allow them to be packaged in flexible, foil-backed polymer laminate casing. Moderate external pressure on LiPo cells can actually prolong cell life by preventing delamination and deformation. (Cannarella, John; Arnold, Craig B. (1 January 2014). "Stress evolution and capacity fade in constrained lithium-ion pouch cells". Journal of Power Sources. https://spikelab.mycpanel.princeton.edu/papers/103.pdf)
Lithium Battery Electrochemistry
There are many complicated details to electrochemistry of these batteries; this is literally the topic of thousands of doctoral dissertations. For purposes of understanding battery swelling, the electrochemistry can be vastly simplified. Regardless of the electrolyte (Li+ or LiPo) or specific electrode materials, lithium batteries generally work with a similar electrochemistry based on oxidation at the negative-electrode which produces lithium ions and electrons. The lithium ions migrate through the electrolyte to the positive-electrode where they recombine through a reduction reaction with the electrons which were conducted through an external electrical circuit. The simplified and generalized electrochemical reaction is shown below. The specific negative-electrode and positive-electrode materials can be substituted without loss impacting further discussions. LiC6+CoO2C6+LiCoO2
where left to right is discharging and right to left is charging. This reaction is split between the negative-electrode and positive-electrode in the cell. At the positive-electrode: CoO2+Li++e-LiCoO2 and at the negative-electrode: LiC6C6+Li++e-
An important note is that none of these reagents are gaseous. That is, nothing in the normal operation appears to be able to cause battery swelling. There are small volumetric changes during charge or discharge cycles (reference), but these changes do not result in catastrophic failures.
Several side reactions can occur during overcharging (LiCoO2 -> Li+ + CoO2 + e-) or over-discharging (Li+ + e- + LiCoO2 -> Li2O + CoO). These can lead to permanent degradation of the electrode materials and reduce the capacity or energy of the cell. However, the reactions generally do not result in gas formation or battery swelling.
Boundary Issues
The first-order approximation of the lithium battery electrochemistry did not yield a gas generator. As such, the cause of battery swelling must be in a more subtle aspect of the reactions. The interfaces between the electrodes and electrolyte hold many complex interactions. This boundary, commonly referred to as the solid electrolyte interface (SEI), is where lithium ions transition between the electrode and electrolyte. SEI is where electrolyte decomposition happens.
Impact of a swollen battery
Battery swelling is a common problem encountered by all users at some point. This occurs when gas is generated inside the battery due to the breakdown of the electrodes or the electrolyte. In particular, the standard cell voltages at the negative-electrode can readily lead to reductive decomposition of the electrolyte.
This gas generation can cause the battery to expand and deform, leading to potential safety hazards and reduced performance.
Let's talk more in-depth about the effects of swollen batteries on mobile devices.
Effects of battery swelling on mobile devices
Battery swelling has become a major issue for modern mobile devices, causing performance and device degradation and posing safety risks.
Battery swelling, also known as battery bulging, is a common problem in lithium-ion batteries. It is typically caused by the accumulation of gas within the battery, which creates pressure and causes the battery to expand. This can happen for several reasons:
- Overcharging: When a lithium-ion battery is overcharged, it can cause the electrolyte to break down and release gas, which can lead to swelling.
- Overheating: Lithium-ion batteries can also swell if they become too hot. High temperatures can cause the electrolyte to break down and release gas, which can lead to swelling.
- Age: As lithium-ion batteries age, the materials inside can break down, which can cause gas to be released and lead to swelling.
- Mechanical damage: Any damage to the battery, such as dropping it or puncturing it, can cause the electrolyte to leak and create gas, which can lead to swelling.
Battery swelling is not only a cosmetic issue, but it can also cause the battery to malfunction or even fail completely. It is important to take proper care of your lithium-ion batteries, including avoiding overcharging, overheating, and physical damage, in order to prevent swelling and ensure the longevity and safety of the battery.
Additionally, when left unchecked, swollen and abnormal batteries present a real fire hazard, like in the Samsung Note 7 scandal in 2017.
As such, users should take steps to keep an eye out for a bulging or swollen battery, by regularly inspecting their device and opting to replace their battery at the earliest sign of swelling.
Notable Battery Swelling Incidents
The most notable incidents related to battery swelling are recent. Let's cite 2 major issues that happened in 2021.
Microsoft Surface Pro and Surface Book's batteries swelled at a dramatically high rate, causing issues on consumers' devices.
Engineering.com reports 257 cases of swollen batteries, with users having their screens curbed, their chassis broken, and even a burned battery.
Another incident occurred with Apple devices. An Apple customer reported that her iPhone 12's battery swelled, eventually pushing the screen forward.
Despite the battery being sold with the device being faulty, the repair wasn't included in the warranty, and the consumer eventually had to spend over $400 to get it fixed. Needless to say, this is a typical example of an issue caused by battery swell that could have been avoided for the consumer.
Mitigation Techniques
To prevent a battery from getting swollen and minimize its impact on device performance, various techniques have been discovered over the years.
In this section, we will discuss these techniques in detail, including measures that can be taken during the design, testing standards, and manufacturing of batteries.
Existing mitigation techniques
Before covering mitigation techniques, let's list them before, and we will go into more detail after that:
- Safety mechanisms
- Overcharge protection
- Temperature monitoring and management
- Safety vents
Safety mechanisms
Safety mechanisms involve installing mechanisms within the battery and in the device charging circuitry itself to protect against extreme conditions and potential problems that could arise.
These include monitoring charges, automating shut-off if dangerous thresholds are reached, full circuit, and employing layers of physical protection with insulation materials.
Overcharge Protection
One of the most effective ways to prevent battery swelling is to use overcharge protection. This refers to a system that prevents a battery from being charged beyond its maximum capacity.
This can be done using a charger that cuts off when the battery reaches its maximum voltage or by using a software management system that monitors the battery voltage and stops charging when the maximum voltage is reached.
Acroname’s Programmable USB Hubs (such as the USBHub3+ or USBHub3c) are ideal for this purpose. Through a simple software interface, lab technicians can set charging schedules and monitor for odd behavior in device batteries or charging behavior.
Temperature monitoring and management
Another way device labs prevent battery swelling is to monitor and control the temperature of the battery. Battery swelling is more likely to occur at high temperatures, so it is important to keep the battery temperature within the standard operating range.
Safety vents
Safety vents are tiny channels located inside a mobile device's battery casing that allows for airflow and help manage pressure and heat build-up in the batteries that lead to swell.
Thanks to their affordable cost and great prevention power, safety vents are a popular choice among manufacturers when it comes to managing battery swelling issues.
In addition, they can increase the life cycle of the mobile device by preventing unnecessary damage to its internal components of the device due to swelling.
Practices to prevent a swollen battery
Monitoring battery health is key for any electronic device in a laboratory, whether it's a phone, laptop, or another digital device.
Correctly charging is essential – Use a high quality charger that is specifically designed and rated for your device. Cheap chargers can cause issues over the long term when used in a device lab setting.
Also, unplugging it when the battery pack is full is a great idea - according to The Washington Post, it's a great way to increase the battery pack's lifespan. (Alternately, you can use the Acroname hubs to disconnect power without physically unplugging the device, giving you the same result without the manual steps of physically unplugging the device)
Prolonged exposure to heat and cold temperatures can also cause premature wear and tear, storing devices in a cool, dry environment and, where possible, away from direct sunlight.
Mitigation strategies' effectiveness and impact
The effectiveness of different battery swelling mitigation techniques varies and can have an impact on device performance.
Safety mechanisms, such as overcharge protection circuitry or temperature monitoring and management, are the most effective methods.
Overcharge protection systems prevent a battery from being charged beyond its maximum capacity, which can help reduce the risk of swelling caused by overcharging.
Temperature monitoring and management help to keep the battery temperature low in order to reduce the likelihood of a swollen battery.
Safety vents provide a way to manage pressure and heat build-up in the batteries, which helps to further reduce risks.
When using these mitigation techniques, there is often an effect on device performance. For example, overcharge protection systems may limit how quickly a device can be charged, which could have an impact on charging speed.
Additionally, this battery management system can cause devices to run slower than usual as they work to keep temperatures low while still providing power efficiently.
Finally, safety vents allow for airflow but could also decrease waterproofing capabilities in some cases. Each of these mitigation techniques has its own benefits and drawbacks when it comes to impacting device performance. And for this reason, there are recent, promising developments in the battery system.
Developments in battery technology
Recent developments in battery systems have been focused on mitigating swelling, which can lead to reduced performance and even safety hazards.
Three promising solutions are:
- Solid-state batteries
- Self-healing batteries
- Lithium-Sulfur (Li-S) batteries
Solid-state batteries
Solid-state batteries use a solid electrolyte instead of a liquid one, which reduces the cells' risk of swelling and increases energy density.
Self-healing batteries
Self-healing batteries are designed to repair themselves when damaged, increasing their lifespan and reducing the need for replacements.
One emerging technology in this field is based on advanced sensing approaches with incorporated sensors as a part of battery cells. Algorithms and advocates work together to detect and repair any damage that may occur within the battery cell.
Ultimately, this would help with regular problems like battery swell.
Lithium-Sulfur batteries
Lithium-Sulfur batteries, on the other hand, use no host structures for storing lithium ions but, instead, consume the graphite anode and transform sulfur during discharge.
The advantage of these batteries is that they have high energy density and long lifespan and don't self-discharge, like liquid-based Li-S batteries. This, in turn, prevents users from charging and discharging the battery pack often and, therefore, avoids battery swelling.
Device Labs
Software developers use device labs to test applications across a wide range of mobile devices and to ensure stability in their products.
In fact, testing real devices, compared to simulations, dramatically increases the chances of delivering and launching a stable and working product out of the lab.
However, one major concern with these labs is the possible swelling of the device batteries, resulting from a chemical reaction between the components inside the battery. This can lead to pressure being formed on the rigid housing of the device, potentially causing it to either catch fire or explode.
To reduce this risk, power sources from host computers must be provided, only allowing data connections once a power source is presented. But that is far from easy, hence why battery swell can still be an issue today.
Acroname Solutions
Acroname's solutions allow software developers to better understand and address battery swelling issues in mobile devices.
Our tools offer real-time telemetry data on voltage, current, and power measurements to detect and proactively manage the issue before it can become hazardous. This helps to identify any potential failure in a cell level or battery's ability to take charge or discharge and provides guidance for controlling or limiting charge cycles on the battery systems of a device.
By enabling engineers to measure the current at ports, they can observe when battery values are lower than expected due to swelling. On top of that, Acroname's hubs enable engineers to estimate the internal resistance of a battery so they can identify when servicing should be performed and prevent further issues.
In short, our hubs make it easy to quickly enable and disable ports, simulating the effects of removing power from a mobile device.
Our USBHub3c enables a breakthrough in powering mobile devices through dynamic power negotiations previously unobtainable with traditional USB interfaces.
Through this, they can make sure the device is not overcharged and that the necessary power supplied is consistent in order to protect the battery system from overcharging.
Overall, Acroname offers solutions that allow brands and software developers to better understand the health of their batteries.
Conclusion
As mobile device technology advances, battery swelling remains a major issue. To ensure the overall safety and longevity of our devices, it is important to be proactive in understanding how batteries work and monitoring their health.
Acroname provides innovative solutions that allow software developers to better understand and address battery swell issues in mobile devices with real-time telemetry data.
These tools make it easy for engineers to detect any potential failure before they become hazardous and provide guidance for controlling or limiting charge cycles on a device.
With these powerful solutions from Acroname, we can stay ahead of the curve when it comes to protecting our mobile devices from battery swell problems.
References
Vetter, J.; Novák, P.; Wagner, M.R.; Veit, C. (9 September 2005). "Ageing mechanisms in lithium-ion batteries". Journal of Power Sources. (https://las493energy.files.wordpress.com/2014/11/2005_vetter_jpowsourc.pdf)
Roman Imhof and Petr Novák 1999 J. Electrochem. Soc. 146 1702 (https://pubs.rsc.org/en/content/articlehtml/2021/cp/d1cp00359c)
Add New Comment